Lateral-torsional buckling is a critical phenomenon in structural engineering that affects unrestrained beams under certain load conditions. This type of buckling occurs when a beam subjected to bending experiences both lateral displacement and twisting. In essence, the beam fails not just by bending, but by a combination of bending, lateral displacement, and rotation, which can significantly impact the stability and safety of structures. This article explains the causes, effects, and factors influencing lateral-torsional buckling, providing a deeper understanding of how to prevent it in engineering designs.
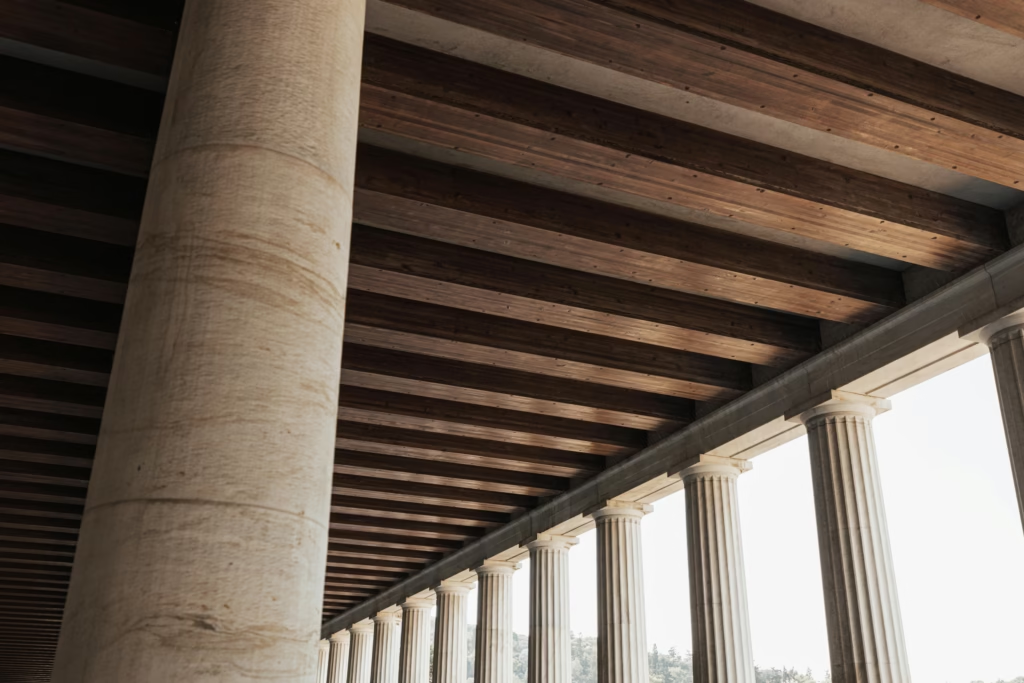
I. Introduction to Lateral-Torsional Buckling
Lateral-torsional buckling is commonly observed in beams that are not adequately restrained, particularly steel beams. Unlike simple bending, which involves a beam flexing along its length, lateral-torsional buckling involves more complex deformations, where the beam not only bends but also twists and shifts laterally. The compression flange of the beam moves laterally, while the tension flange resists the displacement, attempting to keep the beam straight. This phenomenon can lead to premature failure if not properly accounted for in the design of structures.
II. Causes of Lateral Deflection in Beams
A. Unrestrained Beams
Lateral-torsional buckling typically occurs in unrestrained beams. These are beams where the compression flange is free to move laterally and rotate without significant support or restraint. When a load is applied to the beam, the compression flange tries to move laterally in response to the forces, while the tension flange works to resist this deflection and maintain the beam’s straightness.
B. Lateral Movement and Its Effects
As a load is applied to the beam, the compression flange experiences a lateral displacement, while the tension flange resists this movement. This creates a complex interaction between the two flanges. The compression flange’s attempt to deflect laterally creates internal forces, and these forces give rise to restoring forces that act to resist the lateral bending. However, the restoring forces alone are not sufficient to prevent buckling. The lateral component of the tensile forces from the tension flange, in combination with the restoring forces, helps to resist buckling. This interaction between restoring forces and tensile forces is critical to understanding the beam’s resistance to lateral-torsional buckling.
III. Causes of Torsional Effect in Beams
In addition to lateral displacement, the forces acting within the flanges of the beam also cause it to twist about its longitudinal axis. This twisting, or torsional effect, is another crucial component of lateral-torsional buckling. The beam’s resistance to this twisting is governed by the torsional stiffness of the beam section, which is influenced by the thickness of the beam’s flanges.
A. Torsional Resistance and Flange Thickness
A beam’s ability to resist torsion depends largely on the thickness of its flanges. Beams with thicker flanges have a greater resistance to twisting compared to those with thinner flanges. This is because thicker flanges provide higher torsional stiffness, improving the beam’s overall bending strength and reducing its susceptibility to lateral-torsional buckling. Conversely, beams with thinner flanges are more prone to twisting and may buckle more easily under load.
IV. Factors Affecting Lateral-Torsional Buckling in Beams
Several factors influence how prone a beam is to lateral-torsional buckling. These factors include the location of the applied load, the shape of the bending moment along the beam, and the conditions of the end supports. Let’s explore each of these factors in detail.
A. Location of the Load Applied
The position of the load relative to the beam’s shear center plays a significant role in determining the beam’s susceptibility to lateral-torsional buckling. The shear center is the point through which the shear force must act to avoid inducing any twisting of the beam. When a load is applied directly at or below the shear center, the beam is less likely to undergo lateral-torsional buckling.
However, if the load is applied above the shear center, the forces can cause the beam to twist and laterally deflect, increasing the risk of buckling. Loads applied above the shear center are referred to as destabilizing loads, as they destabilize the beam and make it more susceptible to lateral-torsional buckling. On the other hand, loads applied at or below the shear center are called non-destabilizing loads and do not significantly contribute to buckling.
B. Shape of the Bending Moment
The distribution of the bending moment along the length of the beam also affects its resistance to lateral-torsional buckling. A uniform bending moment, where the moment is constant across the beam’s length, typically results in lower resistance to buckling. In contrast, a beam subjected to varying bending moments along its length, such as those that occur with point loads or moment variations, is more likely to have a higher buckling resistance.
The varying moments create a more complex load distribution that can reduce the chances of buckling in certain sections of the beam. Understanding the bending moment distribution is essential for predicting and mitigating the risk of lateral-torsional buckling in beams.
C. End Support Conditions
The type of end supports and the degree of restraint they provide also influence the buckling resistance of a beam. A beam that is more restrained at the ends—such as one with fixed or clamped supports—has a greater resistance to lateral-torsional buckling because the supports prevent excessive movement of the beam. On the other hand, beams with less restrained end supports, such as simply supported or free ends, are more susceptible to buckling due to the lack of resistance to lateral displacement and rotation at the beam’s ends.
Increasing the level of restraint at the supports can significantly enhance the beam’s stability and reduce the risk of lateral-torsional buckling, making it a key consideration in the design of structural systems.
V. Conclusion
Lateral-torsional buckling is a complex failure mode that can occur in unrestrained beams when they are subjected to bending loads. It involves both lateral displacement and twisting of the beam, and its occurrence depends on several key factors, including the location of the applied load, the shape of the bending moment, and the end support conditions. To prevent lateral-torsional buckling, it is crucial to understand these factors and design beams with appropriate restraints, load applications, and flange thicknesses. By addressing these elements, engineers can ensure the stability and safety of structures, avoiding the risks associated with lateral-torsional buckling and optimizing the performance of beams in various applications.